A Beginner’s Guide to Understanding Quantum Dots
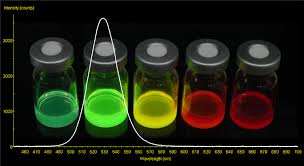
Quantum Dots are a technology that have been gaining attention lately. They are being used to develop sensors that are able to detect chemical and biological warfare agents. In addition, they are also being tested as sensors for neuroscience.
InP is the base chemistry of choice for quantum dots
Quantum dots are nanoparticles containing a core-shell structure. The nanoparticles have unique properties and electronic characteristics. Typical quantum dots are used for cellular interfacing and light-harvesting applications.
The chemistry of the quantum dots has been investigated, and a number of techniques have been developed to produce the dots. Among them are colloidal synthesis and electrochemical assembly.
For instance, a colloidal synthesis technique is known as the magic size cluster. It is a simple process that produces a narrow quantum dot. This method has several advantages, such as high efficiency and a wide range of downstream applications.
Another technique, called plasma synthesis, is another cost-effective way to produce the quantum dot. It is also an easy method to use. InP is a promising material for use in a variety of applications, including light-harvesting. However, it has not been widely studied for emissive applications.
InP quantum dots can be obtained by heating a solution containing the precursors In and P. A yellowish green color indicates the presence of the compounds, and the color of the reaction solution can be observed visually.
QDs behave in a similar way to atoms
Quantum dots are semiconductor nanocrystals that have unique properties. In the simplest case, they contain a semiconductor shell and a semiconductor core. The core is a discrete energy level. It is possible for an excited electron to jump to a higher energy level and subsequently release energy as a photon.
Electronic excitation in QDs is resolved by the surrounding dielectric. This leads to a tunable emission spectra. Depending on the size and composition of the QD, it can be produced in various wavelength ranges. Smaller quantum dots emit higher frequencies, while larger ones produce lower frequencies.
Quantum dots are used in bioimaging applications due to their ability to absorb light and to excite electrons. They are also very useful for high-throughput screening, fluorescence multiplexing, and single-molecule tracking. These advantages also make them suitable for high-resolution cellular imaging. However, there are a few disadvantages.
One major disadvantage is the relatively low stability of QDs. Although there are new generations of QDs with alloyed composition gradients, their large size has been known to cause problems. Another issue is their inability to perform adequately as acceptors in FRET reactions. A number of studies have focused on the mechanism of QD cytotoxicity.
Electron transfer in quantum confinement has lower reorganization energies than molecular systems. This has led to a more robust violation of the Marcus hypothesis. Nevertheless, the kinetic energy imparted by quantum confinement is larger than the Coulomb binding energy.
QDs can be used as neuroscience sensors
Quantum Dots (QDs) have a unique set of fluorescence properties and can be used in many different applications, including neuroscience. They have a broad excitation spectrum and narrow emission spectra, high fluorescence lifetimes, and a large size range. These attributes make them excellent probes for bioimaging. However, there are some downsides to using QDs in these applications.
One of the most important disadvantages to using QDs is the risk of exposure to toxic chemicals. Many of the current materials that are used to produce them pose a serious health threat.
Fortunately, new generations of QDs offer a wide variety of potential applications. These include single-molecule studies, diagnostics, tumor targeting, and in vivo observation of cell trafficking.
Other advantages of using QDs in biological sensors include a very wide range of fluorescence spectra, excellent photostability, and narrow emission spectra. These characteristics are beneficial for tracking membrane receptors and regulatory proteins, and long-term labeling of endosomes.
In addition, novel QDs with an alloyed composition gradient can display continuously strong fluorescent emissions without “blinking” behavior. This is because electron-hole pairs in larger dots have longer lifetimes. Therefore, their photoelectrical response is much better than that of lower-energy organic dyes.
QDs are making waves in the world of TVs and monitors
Quantum dots are making a splash in the TV and monitor industry. They are being referred to as the next big thing. With their promise of enhanced color quality, they are being applied in televisions and other displays.
The name QD is a play on the word “quantum” in the context of nanocrystal particles. As opposed to conventional LEDs, which are white in color, quantum dots are able to absorb a broad spectrum of light.
While the QD is still in its infancy, newer versions are already being used for applications such as single molecule studies and tumor targeting. There is also potential for QD-based color filters that may make their way into displays in the near future.
Quantum dots are an interesting and versatile material that can serve a wide range of applications. From photo-emitting diodes to image sensors to spin-coating, they are found in a variety of applications.
A quantum dot is a semiconductor nanocrystal that has trapped electrons. These nanoparticles have a small size (a few nanometers in diameter) and can be chemically synthesized or fired at a substrate using a laser. In addition to their light-emitting properties, they can also be used for their electrical gating ability.
Quantum dot technology has increased in popularity over the past few years. The latest quantum dot innovation, QLED, uses a layer of color-reactive quantum dots to replace the OLED display in a television.
QDs are being tested as sensors for chemical and biological warfare agents
Quantum Dots (QDs) have a high extinction coefficient and wide absorption spectra, making them an ideal candidate for biochemical and microbial detection. They also have a low photobleaching, making them a promising candidate for molecular cellular imaging. QDs can be used for targeted drug delivery, in vivo fluorescent tagging, tumor tracing and therapy X-ray beam location.
Chemical and biological warfare agents pose a real threat. Biological agents are contagious and can spread rapidly, require minimal resources to survive and replicate rapidly. However, they are usually invisible when they first arrive and are therefore hard to detect.
One important class of potential bioweapons are soluble protein toxins. These toxins can be detected by detecting their presence or sequenced from their RNA. Soluble protein toxins are important for a variety of applications, including a wide range of diagnostics. In addition, they can be used to help develop new therapies.
Quantum Dots are relatively inexpensive to manufacture. They can be used to create multiplexed immunoassays. Unlike dye-based methods, QD probes can provide a dynamic range of several orders of magnitude. This is a significant advantage over traditional dye-based methods.
Another application for QDs is in neurology. Researchers can use quantum dots to color code cells and visualize molecular surfaces. It is possible to create a fluorophore that distinguishes normal cells from cancer cells.
Thanks for visiting theomnibuzz